Effects of natural surfactants on the spatial variability of surface water temperature under intermittent light winds on Lake Geneva
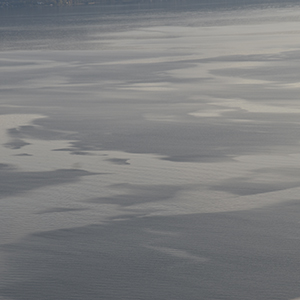
Accepted: 31 May 2022
HTML: 125
All claims expressed in this article are solely those of the authors and do not necessarily represent those of their affiliated organizations, or those of the publisher, the editors and the reviewers. Any product that may be evaluated in this article or claim that may be made by its manufacturer is not guaranteed or endorsed by the publisher.
Authors
The spatial variability of lake surface water temperature (LSWT) between smooth and rough surface areas and its potential association with the natural surfactant distribution in the surface microlayer were investigated for the first time in a lake. In spring 2019, two different field campaigns were carried out in Lake Geneva to measure: i) the enrichment factor of fluorescent dissolved organic matter (FDOM) as a proxy for biogenic surfactants, and ii) LSWT and near-surface water temperature profiles while simultaneously monitoring water surface roughness in both cases. Results indicate that, under intense incoming short-wave radiation and intermittent light wind conditions, the atmospheric boundary layer (ABL) was stable and the accumulation of heat due to short-wave radiation in near-surface waters was greater than heat losses by surface cooling, thus creating a diurnal warm layer with strong thermal stratification in the water near-surface layer. A threshold wind speed of 1.5 m s-1 was determined as a transition between different dynamic regimes. For winds just above 1.5 m s-1, the lake surface became patchy, and smooth surface areas (slicks) were more enriched with FDOM than rough areas (non-slick) covered with gravity-capillary waves (GCW). Sharp thermal boundaries appeared between smooth and rough areas. LSWT in smooth slicks was found to be more than 1.5°C warmer than in rough non-slick areas, which differs from previous observations in oceans that reported a slight temperature reduction inside slicks. Upon the formation of GCW in non-slick areas, the near-surface stratification was destroyed and the surface temperature was reduced. Furthermore, winds above 1.5 m s-1 continuously fragmented slicks causing a rapid spatial redistribution of LSWT patterns mainly aligned with the wind. For wind speeds below 1.5 m s‑1 the surface was smooth, no well-developed GCW were observed, LSWT differences were small, and strong near-surface stratification was established. These results contribute to the understanding and the quantification of air-water exchange processes, which are presently lacking for stable Atmospheric Boundary Layer conditions in lakes.
Edited by
Marco Toffolon, Department of Civil, Environmentaland Mechanical Engineering, University of Trento, ItalyHow to Cite

This work is licensed under a Creative Commons Attribution-NonCommercial 4.0 International License.
-
Seyed Mahmood Hamze-Ziabari, Mehrshad Foroughan, Ulrich Lemmin, David Andrew BarryRemote Sensing : 2022
-
Mehrshad Foroughan, Seyed Mahmood Hamze‐Ziabari, Ulrich Lemmin, David Andrew BarryGeophysical Research Letters : 2022